What is a Calorimeter Used For?
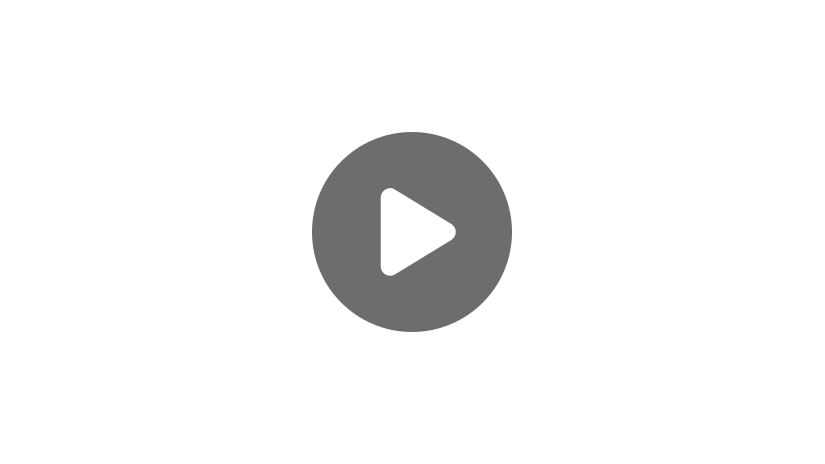
Hi, and welcome to this video on calorimeters, the experimental tool chemists use to measure the heat released or absorbed by a chemical or physical change. Today, we’ll focus on how to use a calorimeter to measure the specific heat capacity of a substance and highlight a common source of error in experiments. Let’s get started!
Let’s start with a quick review of heat capacity, so we actually know why we need a calorimeter.
Measuring Specific Heat Capacity
The heat capacity is the quantity of heat (thermal energy, measured in Joules) required to raise the temperature of a substance by 1 Kelvin. The specific heat capacity is the heat capacity per gram of the substance and molar heat capacity is the heat capacity per mole of substance. Heat capacities vary across materials and depend on the specific molecular structure and internal interactions of the substance. It’s an important thermodynamic quantity and is related to both entropy and enthalpy.
And as mentioned, we measure heat capacities using calorimeters.
The general schematic of a calorimeter is a well-insulated vessel for which we know the heat capacity; in other words, it’s calibrated. So when the temperature of the calorimeter increases, we can equate that to an amount of heat absorbed or released using the calorimeter’s heat capacity in this equation:
q, the heat absorbed/released by the calorimeter is equal to the heat capacity of the calorimeter times the change in temperature.
So, if we add something to the calorimeter that releases heat, we can use the calorimeter’s change in temperature to calculate how much heat was released. For example, if we know the calorimeter needs 100 Joules for the temperature to increase by 10 K, and we add a substance that raises the temperature by 10 K, we then know that the substance must have released 100 Joules of thermal energy.
And provided that we know the mass and temperature change of the substance, we can calculate its specific heat capacity.
The heat capacity of the unknown is equal to change in heat of the calorimeter divided by the mass of the unknown times the change in the temperature of the unknown.
\(C_{\text{ }unknown}=\frac{q_{cal}}{\text{mass}_{\text{ }unknown}\times \Delta T_{\text{ }unknown}}\)
Okay, let’s move away from this generic/theoretical calorimeter and consider a real calorimetry experiment—one that you could even set up at home if you wanted.
To start, you need two nested Styrofoam cups, which are used to create what is aptly named a coffee-cup calorimeter. The top is fitted with a cork stopper, which allows for a thermometer and stirrer to enter.
It’s filled with 100 g of room temperature water, 298 K. The heat capacity of water is well known to be 4.184 Joules per gram Kelvin. We add in a piece of aluminum that weighs 12.5 grams, which was just heated in boiling water, so it has a temperature of 100 °C or 373 K. The aluminum releases heat which is then absorbed by the water until both water and aluminum reach thermal equilibrium. Using the thermometer, we see that the temperature of the water has increased from 298 to 299.7 K, which means the aluminum decreased from 373 K to 299.7 K. And with this information, we can now calculate the specific heat capacity of aluminum.
Let’s start by calculating how much thermal energy was absorbed by the water using the following equation. The heat absorbed is equal to the specific heat capacity of water times the mass of water times the change in temperature. Plugging those values in, we find that the water absorbed 711 Joules of thermal energy.
\(q_{\text{ }{water}}=c_{\text{ }water}\times mass_{\text{ }water}\text{}\times \Delta T_{\text{ }water}\)
\(q=4.184\text{ }\frac{J}{g\cdot K}\times 100\text{ g}\times (299.7\text{ K}-298\text{ K})=711\text{ J}\)
We’re going to assume that all that thermal energy came from the piece of hot aluminum, so we can use that value to solve for the specific heat capacity of aluminum.
In other words, we’re saying that there is no exchange of heat or work from our system to the surroundings. So, all of the heat released from the aluminum was transferred to the water and the only heat transferred to the water was from the aluminum.
We can represent this mathematically by saying that the heat released by the aluminum is equal to the negative of the heat absorbed by the water.
\(q_{\text{ }water}=-q_{\text{ }aluminum}\)
Now, we can set up an equation to solve for the specific heat capacity of aluminum, where it equals the negative of the heat absorbed by the water divided by the mass and temperature change of the aluminum. Remember that change in temperature is always final minus initial, which for the aluminum, gives us a negative value and cancels out the negative for the 711 Joules. If you come up with a negative value for a specific heat capacity, you’ve made a mistake somewhere, so make sure to keep track of your minus signs.
\(-711\text{ J}=C_{\text{ }aluminum}\times mass_{\text{ }aluminum}\times \Delta T_{\text{ aluminum}}\)
\(C_{\text{ }aluminum}=\frac{-711\text{ J}}{mass_{\text{ }aluminum}\times \Delta T_{\text{ }aluminum}}\)
\(C_{\text{ }aluminum}=\frac{-711\text{ J}}{12.5\text{ }grams\times (299.7\text{ K}-373\text{ K})}\)
\(C_{\text{ }aluminum}=0.776\frac{ J}{g\cdot K}\)
So, our experimental value for the specific heat capacity of aluminum is 0.776 Joules per gram Kelvin. Let’s compare this to the literature value (the highly accurate value determined by chemists). The literature value is 0.900 Joules per gram Kelvin. Our experimental value is certainly in the ballpark, but is still ~14% off. Why is that?
Let’s consider where the error could have come from. Let’s assume we measured and weighed the water and aluminum accurately and that the specific heat capacity of water is correct (which it is). That leaves the temperature measurement. Let’s also assume we read the thermometer correctly, so why was our value still wrong? The error lies in our assumption that all of the heat released by the aluminum stayed in the system. Some of the heat was transferred to the Styrofoam and into the lid and out into the surroundings. Notice that our “calorimeter”, the part of our system that’s calibrated, was simply the water. We weren’t tracking the temperature increase in any other part of the system, like the Styrofoam, stirrer, or cork lid. Thus, the rise in temperature we measured was probably a little low and did not reflect all the heat released from the aluminum.
We can improve the accuracy of our measurement by using a more sophisticated calorimeter that reduces the transfer of heat out of the system. Here, we have an insulated double wall calorimeter with a fitted top. Let’s repeat the experiment using this setup and see how it changes the results.
With the exact same experimental setup, we find that with our new calorimeter, the temperature of the water reached 299.9 K. So now let’s see how that adjusts our calculation of the specific heat capacity for aluminum. Since this is just like the previous calculation, pause the video and give it a try yourself!
Alright. Let’s see how that small change in temperature impacted the result. We still begin by calculating the amount of heat absorbed by the water, but now the final temperature is 299.9 K instead of 299.7 K, which gives us 795 Joules. Now, plug that value into the equation for the aluminum heat capacity. Remember, it should be -795 Joules and you need to change the final temperature here as well. Now, our experimental value is 0.870 Joules per gram Kelvin.
\(q_{\text{ }water}=c_{\text{ }water}\times mass_{\text{ }water}\times \Delta T_{\text{ }water}\)
\(q_{\text{ }water}=4.184\frac{J}{g\cdot K}\times 100\text{ g}\times (299.9 \text{ }K-298\text{ }K)=795\text{ }J\)
\(-795\text{ }J=c_{\text{ }aluminum}\times mass_{\text{ }aluminum}\times \Delta T_{\text{ }aluminum}\)
\(C_{\text{ }aluminum }=\frac{-795\text{ }J}{mass_{\text{ }aluminum}\times \Delta T_{\text{ aluminum}}}\)
\(C_{\text{ }aluminum}=\frac{-795\text{ }J}{12.5\text{ }grams\text{ } \times (299.9\text{ }K-373\text{ }K)}\)
\(C_{\text{ aluminum}}=0.870\frac{J}{g\cdot K}\)
This is only 3% off the literature value, suggesting that this calorimeter released less heat into the surroundings, providing us with a more accurate result. Also, notice that it was a difference of 0.2 K between the two final temperatures measured, so these are small changes that make significant impacts on our results.
Notice that even in the more advanced calorimeter, we are still only using the water as the calibrated portion, we’re just doing a better job of limiting heat loss. Consequently, there are even more sophisticated calorimeters in which you can measure the heat capacity of the entire vessel rather than just the water, which means we can reduce our measurement error even further. A popular setup is the bomb calorimeter, which functions at constant volume, rather than constant pressure like the two models we demoed today. Along with measuring the heat capacity of substances, calorimeters can be used to measure the heat released or absorbed during chemical reactions.
Okay, let’s wrap up by reviewing what we’ve learned. A calorimeter is an instrument for which we know (or can determine) the heat capacity, which means we can calculate the heat released by a sample by measuring the temperature change of the calorimeter using this equation.
\(q_{\text{ calorimeter}}=c_{\text{ calorimeter}}\times mass_{\text{ }calorimeter}\times \Delta T_{calorimeter}\)
For our examples today, the “active” part of the calorimeter was water, which means we dealt with the specific heat capacity and weight of water. But for more complex setups, this will vary.
We then assume that the heat absorbed by the calorimeter was equal and opposite to the heat released by the substance. So by knowing the weight and temperature change of the unknown, we can calculate the unknown heat capacity.
\(C_{\text{ }unknown}=\frac{-q_{\text{ }calorimeter}}{mass_{\text{ }unknown}\times \Delta T_{\text{ unknown}}}\)
As we’ve seen today, calorimeters can be super simple—just water in a cup. But in research labs, they tend to be a bit more sophisticated and are better insulated so as to retain all the heat released.
Now, here’s a practice problem for you before we go.
Practice Problem
Imagine we found a piece of gold, but didn’t know if it was real gold or pyrite, commonly known as fool’s gold. How could we determine the true identity of the substance? Well, it turns out we could figure it out by measuring the heat capacity! The specific heat capacity of gold is 0.129 J/g-1 K-1 and the specific heat capacity of pyrite is 0.386 J/g-1 K-1. Definitely different enough for us to tell the difference.
We first weigh the substance (it’s 21.0 grams), heat it in boiling water, and add it to a coffee-cup calorimeter with 50.0 grams of room-temperature water. The temperature of the water increases to 296 K. Pause the video, calculate the specific heat capacity of the unknown, and determine if it’s fool’s gold or real gold. Start by calculating the heat absorbed by water using this equation.
\(q_{\text{ }water}=c_{\text{ }water}\times mass_{\text{ }water}\times \Delta T_{\text{ }water}\)
Alright, let’s go through the problem together now.
First, we calculate the heat absorbed by the water
\(q_{\text{ }water}=c_{\text{ }water}\times mass_{\text{ }water}\times \Delta T_{\text{ }water}\)
\(q_{\text{ }water}=4.184\frac{ J}{g\cdot K}\times 50.0\text{ }g\text{ }\times (296.0\text{ }K-293.0\text{ }K)=628\text{ }J\)
So the heat absorbed by water is the specific heat capacity of water times the weight of the water times the temperature change, which gives us 628 J. Those 628 J were released by the unknown, so we can set up a new equation to solve for its specific heat capacity.
\(-628\text{ }J=C_{\text{ }unknown}\times mass_{\text{ }unknown}\times \Delta T_{\text{ }unknown}\)
\(-628\text{ }J=C_{\text{ }unknown}\times 21.0\text{ }g\times (296.0\text{ }K-373.2\text{ }K)\)
\(C_{\text{}unknown}=\frac{-628\text{ }J}{21.0\text{}grams \times (296.0\text{ }K-373.2\text{ }K)}\)
The specific heat capacity of the unknown is equal to the heat released by the unknown (which is the negative of the heat absorbed by the water) divided by the weight and temperature change of the unknown (make sure this is final minus initial, which is a negative number and cancels the negative sign upstairs). This gives us a heat capacity of 0.387 Joules per gram Kelvin.
\(C_{\text{}unknown}=0.387\frac{J}{g\cdot K}\)
Alas, our unknown was fool’s gold, not real gold! Well, at the very least, hopefully that calculation went well for you! If it didn’t, keep practicing!
Alright, that’s a wrap for today! Thanks for watching, and happy studying!