Dark Matter
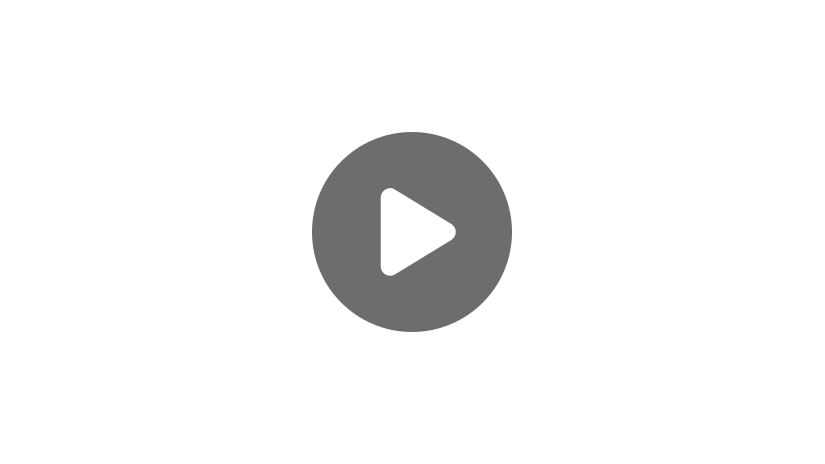
Dark matter is famously impossible to see. How, then, do astronomers know it exists? To understand how astronomers discovered dark matter, first you must understand how astronomers study motion and the kinds of motions astronomers can see in space. For planets in our solar system, we know that some orbit the Sun faster than others. One way to compare the motion of the planets is to construct a graph called a rotation curve. A rotation curve graphs velocity on the y-axis and average distance from the Sun on the \(x\)-axis.
Rotation Curves
To make a rotation curve for the planets of our solar system, astronomers make careful observations and utilize principles like the Doppler effect to get values for velocity and distance.
Once astronomers have the velocity of each planet and its distance from the Sun, it is now possible to plot these on a rotation curve.
The farther a planet is from the Sun, the slower it orbits. Johannes Kepler discovered this relationship for the planets, and Sir Isaac Newton would later explain why: because the Sun’s force of gravity is causing these orbits, planets farther from the source of gravity experience less force and, by extension, less acceleration and thus move more slowly.
Now, since our galaxy has a flat structure with a massive central object that everything orbits, astronomers thought it would be reasonable to plot a rotation curve for stars orbiting the galactic core. Compare, for example, these two artistic expressions. The one on the left is a drawing of the solar system and the one on the right is a drawing of a galaxy.
Due to the similarities between these two systems, astronomers assumed that the rotation curve for our galaxy would look similar to the one for the solar system. If the bright central region of the galaxy contains the source of the galaxy’s gravity, then stars farther from that center will move more slowly. The center of the galaxy contains a supermassive black hole, which is a plausible source for the gravity at the center of the galaxy. When astronomers did plot the rotation curve for the galaxy, however, it was not the curve they expected. The speed of galactic objects increases the farther they are from the center!
This type of rotation curve has been called a flat rotation curve. Recall that fast orbital velocity means an object is close to the source of gravity. And yet in our galaxy, objects very far from the central supermassive black hole are going the fastest. This means that the central black hole cannot be the gravity source for these stars and dust clouds far from the center. There is some other gravity source.
Discovery
In 1933, astronomer Fritz Zwicky was studying the way galaxies moved in clusters. He used the orbits of galaxies to determine the mass of their clusters. He found that each cluster he observed was hundreds of times more massive than expected. Zwicky’s findings seemed to indicate that there is unaccounted-for mass in galaxy clusters and he called this missing mass “dark matter.” At the time, many astronomers questioned his methods and conclusions so Zwicky’s research went ignored for several decades.
Then in the 1970s, Vera Rubin, a pioneering female astronomer, made rotation curves for many galaxies in our sky and showed that they all had similar shapes. It turns out that this is not a problem unique to our galaxy.
After Rubin’s work, astronomers returned to Zwicky’s findings and found that his results were consistent with Rubin’s findings. At this point, scientific interest and investigation in dark matter grew rapidly, with astronomers observing more and more objects and collecting a lot of data trying to confirm or deny the existence of dark matter.
Both Rubin and Zwicky’s methods take advantage of Doppler shifts to determine mass. A different, independent way to measure mass in space is called gravitational lensing. According to Albert Einstein’s Theory of General Relativity, mass bends light. For small masses, this is undetectable. You do not see a flashlight beam warp because you wave your hand in it, for instance. Galaxy clusters are extremely massive, though. Light traveling through a cluster is visibly warped. The more massive the cluster, the more the light bends going through it. Therefore, astronomers can judge the mass of the cluster from how much light is bent by it. Measurements from gravitational lensing of galaxy clusters agree with measurements from Zwicky’s and Rubin’s methods.
Realizing that there was a consensus about the existence of extra matter, astronomers then turned to the question of what it might be. Initially, astronomers assumed it was regular matter. Examples of possible “regular” sources of dark matter include gas and dust. The problem is that both of these emit light in infrared wavelengths. And yet, no infrared glow has been found that corresponds to the locations of dark matter.
The Source of Dark Matter
One possibility is that these sources of dark matter are too small or dim to easily detect in the infrared. If, instead of dust or gas clouds, dark matter were composed of individual small objects far from each other, the brightness of these may be nearly undetectable. This is the hypothesis that dark matter is composed of MAssive Compact Halo Objects (MACHOs). Examples may be rogue planets, failed stars, or black holes. Black holes receive the most attention because their extreme gravity does not let any light escape. A black hole by itself in space is difficult to detect. Nonetheless, black holes have mass, and mass bends light, so black holes could be observed through gravitational lensing.
Unlike entire galaxy clusters, black holes are very small. In order to have light shine through the area of the black hole, a star or distant galaxy has to pass behind the black hole from our point of view. This rare event causes the background object to flash as its light is magnified. This is called a microlensing event. We have detected black holes through microlensing near the outsides of galaxies! But not very many. This is seen as strong evidence that the MACHO hypothesis is not likely. While the methods for detecting MACHOs work well enough, there are simply not very many MACHOs to find—certainly not enough to explain the missing matter seen in rotation curves.
A second hypothesis states that dark matter is not just dark because it is not well-lit or it is glowing subtly. Rather, it is transparent in all wavelengths. Since there is no known kind of large object that fits this description, scientists turned to particle physics. Weakly Interacting Massive Particles (WIMPs) contain mass but only interact with other matter through the gravitational and weak forces, hence the name “weakly interacting.” The only confirmed WIMP is a particle called the neutrino. Neutrinos are very small and do have mass. There are trillions of neutrinos going through your body right now, but to a neutrino, your body looks like empty space. No neutrino touches any part of your body, as they instead soar unimpeded through the space between the atoms that comprise your body. Catching neutrinos is difficult and requires massive detectors such as the IceCube Neutrino Observatory, a square kilometer of Antarctic ice that has been laced with detectors.
In spite of the difficulty, scientists have observed them enough to make measurements, so we know their mass and can estimate how many neutrinos exist in the universe. Unfortunately, neutrinos are not massive enough nor are there enough of them to account for the missing matter observed in galaxies and galaxy clusters. Most astronomers and physicists believe there is another particle responsible for dark matter, one even harder to catch and more massive than neutrinos. This is the hypothesis currently in favor in the scientific community. One way scientists look for candidates for a dark matter particle is by particle collisions manufactured here on Earth. Particle accelerators like the Large Hadron Collider intentionally crash particles together near the speed of light in order to see what they break apart into.
There is one additional possibility—that dark matter doesn’t exist at all. A few researchers question our understanding of gravity and suggest that the equations we know of from Newton and Einstein are not complete or are flawed in some way. This hypothesis is called the MOdified Newtonian Dynamics (MOND) for the idea that we need to modify our understanding of gravity to better explain the universe around us. While MOND researchers insist that their model is consistent with observations, it has not gained much traction. Most dark matter researchers are focused on designing detectors to catch new kinds of particles.
So why is dark matter important? Well, if dark matter exists, then it would account for 83% of all matter in the universe. As for the entire mass-energy budget of the universe, about 27% of it is categorized as dark matter. (About 4% is regular, observable matter and the rest is classified as dark energy—a whole field of study unto itself.) If 27% of your budget were missing, you would certainly care! Galaxies and galaxy clusters may look like glowing masses of stars, but actually they are mostly invisible! From rotation curves, astronomers can see that even at large distances from the center of a galaxy, the amount of dark matter is very high. What does a galaxy look like when you include the dark matter, then?
This large area where only dark matter exists is called a galaxy’s dark matter halo. The light we see coming from a galaxy is only a small portion of the mass contained in a galaxy. One common analogy is that the luminous matter is like light shining on dew drops on a spider web. The dark matter is the underlying web that holds the luminous matter together. This is true on a larger scale as well. Even the universe as a whole is held together by the invisible gravitational web created by dark matter—whatever it is.
That’s all for this review! Thanks for watching, and happy studying!