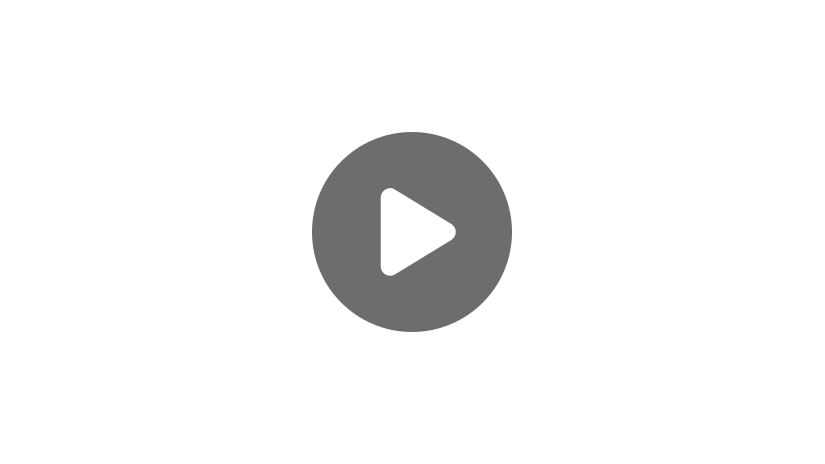
Hi, and welcome to this review of bonding and structure!
In this video, we’re going to take a look at how the bonding and structure of a substance relate to its physical properties.
This particular subject is simultaneously simple and complex. The easy part is that, broadly speaking, we know that the physical properties of a substance are directly related to the bonding and structure on a microscopic level. So things like the melting point, boiling point, vapor pressure, and solubility of a substance can usually be understood by considering the molecular structure and bonding type.
The complex part is that there are different types of bonds and lots of different chemical combinations and there aren’t hard and fast rules that dictate exact trends in physical properties. That’s not too surprising given the huge diversity in materials.
To get started, we’ll review the different types of compounds, then consider a few physical properties and look at them from a molecular perspective. Keep in mind that there will likely be exceptions to all the “rules” I present today. But by the end, hopefully you’ll have developed an intuition for how to assess a material based on its bonding and structure and have a good guess as to its physical properties.
Compound Categories
Let’s begin with a quick review of the four primary categories of compounds:
First, ionic compounds, like sodium chloride, are networks of cations and anions held together by strong electrostatic attractions.
These are strong interactions and are generally pretty hard to break. Ionic compounds come in large arrays of repeating ions and, although written as NaCl, sodium chloride should be considered as a whole unit rather than pairs of ions.
Second, molecular compounds, like water, carbon dioxide, and caffeine, are made of many discrete units of the same individual molecules, which are covalently bonded, but the units are held together by relatively weak intermolecular interactions, like hydrogen bonds and dipole-dipole interactions.
Third, covalent-network compounds, like graphite and diamond (both networks of covalent carbon) are held together by a continuous network of covalent bonds.
This is in contrast to molecular compounds, where the bulk phases are held together by intermolecular interactions. Consequently, covalent-network compounds have very different physical properties from molecular compounds even though both have covalent bonds.
Lastly, metals, like copper or gold, are held together by metallic bonds or what is colloquially referred to as a “sea of electrons”.
The valence electrons of each metal atom are more or less free to swarm around the lattice of metal cations. This unique type of bonding explains a lot about the physical properties of metals, which we will explain as we go.
Melting and Boiling Points
First, let’s consider the melting points for these compounds. The melting point at standard atmospheric pressure will tell us what phase to expect when we encounter these compounds in our daily lives. As your experience might tell you, ionic compounds, covalent-network compounds, and metals typically have melting points well above 25 degrees Celsius.
In other words, all these are usually solids at room temperature. For example, sodium chloride has a melting point of 801 degrees Celsius and quartz melts around 1,670 degrees Celsius. This is a result of the very strong bonds holding the network of atoms together. You need lots of energy to disrupt ionic and covalent bonds, so the melting points are quite high. Metals are also solids at room temperature, with one notable exception being mercury, which melts at -39 degrees Celsius.
Generally, the stronger the bonds holding the atoms together, the higher the melting point. Similar trends in boiling points exist for these compounds, but with much higher temperatures required for vaporization.
Finally, let’s consider the melting points of molecular compounds, things like water, carbon dioxide, and caffeine. As you can tell from our examples, molecular compounds can have very different physical properties. However, they generally have melting points well below those of ionic, covalent-network, and metallic compounds because the molecular units are held together by weak intermolecular interactions. Typically, you can determine relative melting points of molecular compounds by assessing the weight and intermolecular interactions.
Light molecules with weak intermolecular interactions, like carbon dioxide, will have really low melting points and exist as gases at room temperature. Conversely, heavy molecules with significant intermolecular interactions, like caffeine, will have higher melting points and are solid at atmospheric pressures. And then there’s everything in between. Water is light, but has a really strong network of hydrogen bonds so exists as a liquid at room temperature. Basically, to understand molecular compounds, you should always start by assessing their ability to form intermolecular interactions.
Solubility
Now that we’ve considered how bonding and structure impact melting and boiling points, let’s consider another important chemical characteristic—solubility.
As an upfront note, we will talk a lot about water in this section, after all it is the “universal solvent”, but the word solubility doesn’t just mean soluble in water. Solubility is the general measure of a solute’s ability to dissolve in a solvent to form a homogenous solution. While the solvent is often water, it’s important to keep in mind that that’s not always the case.
Alright, let’s dive in.
Polar vs. Nonpolar
When we think about solubility, the phrase, “like dissolves like” often comes to mind. This is most relevant when discussing the solubility of molecular compounds. The “like” in the phrase usually refers to polar or nonpolar molecules, two categories that molecular compounds are broadly split into. Polar compounds have bonds where the electron density is unequally distributed among the atoms. They typically have a lot of heteroatoms (oxygen, nitrogen, halides, etc.), which create areas of positive and negative charge.
Strong intermolecular interactions occur when those areas in one molecule align with the opposite charges in another molecule. Thus, polar molecules tend to dissolve in polar solvents because of these attractive intermolecular forces—the process is energetically favorable. Conversely, nonpolar molecules are more or less neutral throughout and are mostly carbon and hydrogen. This means that they can only form weaker intermolecular interactions with other nonpolar and polar molecules.
Let’s consider an example. When you add oil to water, the water molecules, which are polar and form strong hydrogen bonds, are strongly attracted to each other. While the oil molecules are also attracted to the water molecules, their interactions with water molecules are so weak that they can’t disrupt the network of water molecules and wind up sitting on top of the water. On the other hand, when you add ethanol to water, the two mix because ethanol is also polar and capable of forming hydrogen bonds, and thus can compete with the attractive interactions between the individual water molecules.
What’s particularly instructive about this example of water, oil, and ethanol is that you need to consider solubility from both sides of the equation—the solute and the solvent. The system prioritizes the most attractive interactions. So it’s not that the oil molecules don’t like water; in fact, oil molecules have stronger intermolecular interactions with water molecules than with themselves (which is why a droplet of oil lays flat on a polar surface). It’s that water likes water more than oil.
Similar rules apply to solid molecular compounds. For example, vitamin C is water-soluble with all those highly polar O-H bonds, whereas vitamin D is fat-soluble, with a high concentration of C-H bonds. This results in the two vitamins being absorbed and released from the body in totally different ways.
Solubility of Ionic, Metallic, and Covalent-Network Compounds
Now, let’s consider the solubility of ionic, metal, and covalent-network compounds. Since, for the most part, all of these are solids at room temperature, we’ll just think about their solubility as solids throughout this discussion.
As you’re probably familiar with, some ionic compounds are quite soluble in water. Table salt, for example, dissolves readily in water. However, other salts, like silver chloride, are essentially insoluble in water. Why is that?
First, when salts dissolve, they split into their separate cations and anions. And they do so because of the many ion-dipole interactions between the ions and water molecules. The water molecules form shells around the ions, stabilizing the raw charge. Importantly, salts don’t dissolve because ionic bonds are weak—they are, in fact, very strong as we’ve discussed. When salts dissolve, it’s typically because the interactions with the solvent are, in aggregate, stronger than the ionic bonds.
Another important consideration when thinking about solubility is the change in entropy that accompanies the formation of a solution. Because solutions are generally more disordered than the pure solute and solvent, dissolving a salt usually increases the entropy of the system. Thus, quantitatively, whether or not a salt dissolves is a fine balance between the energy associated with solvating the ions, the strength of the ionic bonds, and the change in entropy.
These finer details we’ll leave for now, but we do want to finish up this discussion with a quick question.
As we know, sodium chloride dissolves readily in water. But, would it dissolve in a nonpolar solvent, like oil? Pause the video and think for a minute.
Hopefully, you answered no. The only reason sodium chloride dissolves in water is because the polar water molecules stabilize the system with many strong intermolecular interactions. Oil molecules, even those with a polar end, aren’t capable of forming enough strong intermolecular interactions to stabilize the sodium and chloride ions. This is actually something you could test at home. Try dissolving salt in olive oil and water. You should see a pretty significant difference!
Covalent-network compounds are generally insoluble regardless of the solvent, as it’s very hard to break the strong covalent bonds, and any resulting ions are too unstable for the solvation energy to make dissolution energetically favorable.
Metals have a wide range of solubility. For example, sodium basically explodes when it’s added to water, lead only dissolves in acidic solutions, and platinum is nearly insoluble in everything save hot aqua regia, a mixture of hydrochloric and nitric acids. The reactivity of metals is connected to their electron configurations.
Electrical Conductivity
Now, since we’ve been discussing the bonding and structure of these four different classes of compounds for quite a while, I want you to pause the video and give your best guess to this question.
Which class of compounds do you think is best at conducting electricity? Keep in mind that the basic definition of electricity is the flow of electric charge. So take a second, and consider your own personal experiences as well as what you’ve learned about the bonding and structure of the different compounds.
Hopefully, you came to the conclusion that metals would be good conductors of electricity—after all, electric wires are made out of metal. We can understand this from our discussion of metal bonding, which we described as a “sea of electrons”. The valence electrons of each metal atom are free to move around, which makes metals great at conducting electricity (and heat!).
Conversely, covalent-network, ionic, and molecular compounds are all generally poor conductors of electricity because the electrons are bound in covalent and ionic bonds and unable to move freely. But if you thought about answering that ionic compounds would be good electrical conductors, you weren’t too far off the mark. When ionic compounds are melted or dissolved in solution, they are great electrical conductors because there can be free movement of charge.
Hardness and Malleability
And lastly, let’s consider how bonding and structure are related to some of the other physical properties of a compound, like how hard or malleable it is. Ionic compounds are hard and brittle. They can’t be molded because if you were to shift the ions, you may align like charges, in which case, the ions would strongly repel each other and break along a clean line.
On the other hand, metals tend to be malleable. The sea of electrons holding them together can shift easily and prevent repulsion between neighboring cations. Consequently, we are able to work metals into complex shapes. Covalent-network compounds are typically hard and unworkable, while molecular compounds run the gamut with their physical properties dictated by the specific intermolecular interactions.
Well, we’ve covered a lot today but have only scratched the surface of how materials connect to their physical properties. Hopefully, you’ve seen that the chemical and physical properties of a compound are always connected to what’s happening at the molecular level. Thus, to best understand material characteristics, we need to know how the atoms are bonded and about the distribution of charge.
That’s it for today. Thanks for watching, and happy studying!